In the realm of electronic circuit design, understanding and effectively managing capacitor ripple currents are essential for ensuring the reliability and performance of electronic systems. In this article, titled “Capacitor Ripple Current Demystified: Causes, Effects, And Solutions,” the complex phenomenon of capacitor ripple current is dissected, offering insight into its causes, effects, and viable solutions.
By delving into this often-overlooked aspect of circuitry, readers will gain a comprehensive understanding of how to mitigate the challenges posed by ripple currents, ultimately optimizing the functionality and longevity of electronic devices.
What Is A Capacitor?
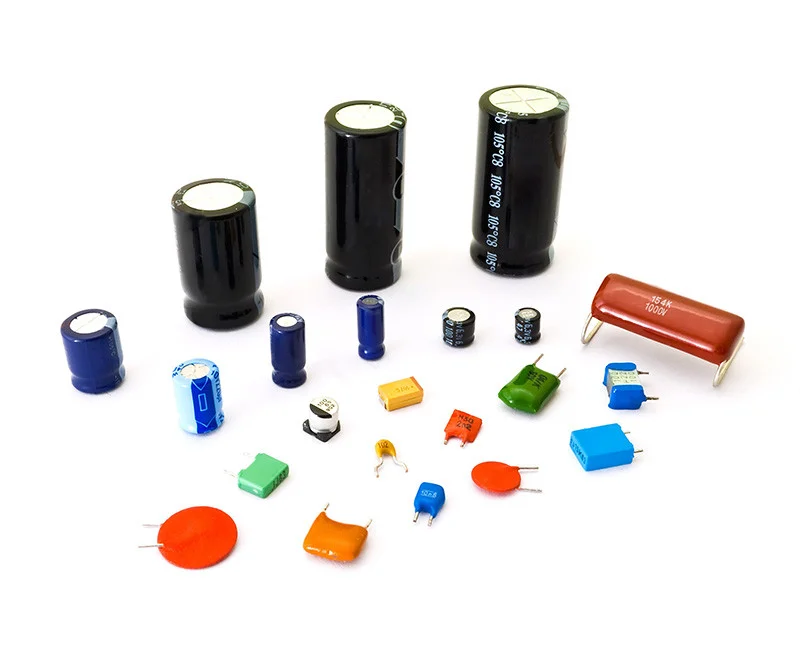
A capacitor is an electronic component that stores electrical energy in an electric field. It consists of two conductive plates separated by an insulating material known as a dielectric. When voltage is applied across the plates, electric charge accumulates on them, creating an electric field between them.
This stored energy can be released when needed, making capacitors useful for various applications such as smoothing power supply voltages, filtering signals, and storing energy in circuits.
Capacitors come in various types and sizes, each designed for specific purposes ranging from small surface-mount capacitors in electronic devices to large electrolytic capacitors used in power electronics.
What Is Capacitor Ripple Current?
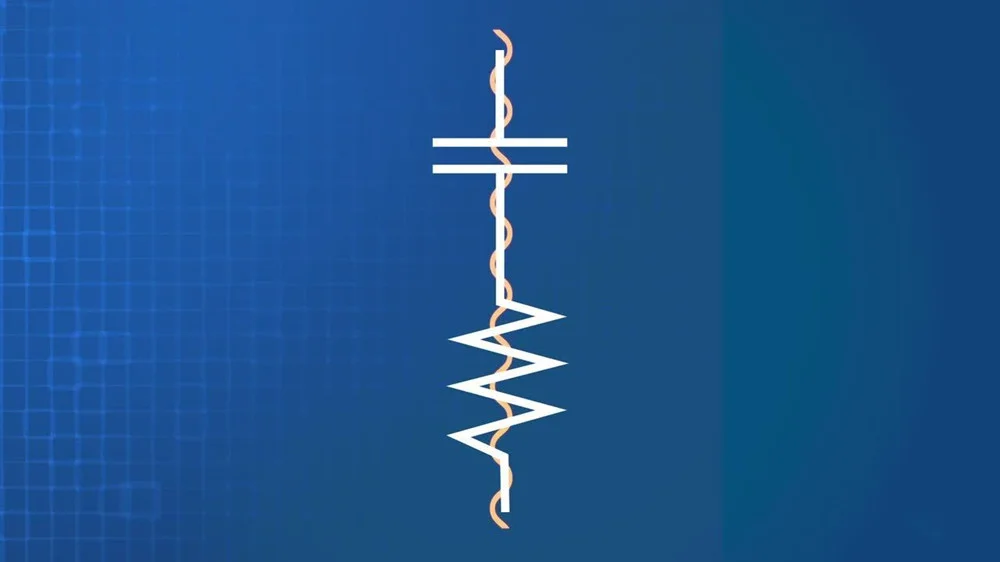
Capacitive filters are essential in power-conversion circuits like AC/DC power supplies, DC/DC converters, and DC links. They counter fluctuations, ensuring stability. Success is typically seen as minimal noise in the DC output and no disturbances in nearby circuitry. Fluctuations overlay stable waveforms, leading to interference from various sources, such as AC rectification.
Switching regulators generate ripple. Capacitors mitigate ripple by absorbing and discharging energy, reducing peaks and troughs. Consequently, capacitors pass ripple current, inducing I2R heating due to Equivalent Series Resistance (ESR). Excessive heating can compromise reliability and reduce component lifetime.
A heatsink may limit temperature rise if space and weight constraints allow. Alternatively, understanding ripple current properties aids in achieving an efficient solution. Capacitor datasheets provide a ripple current rating as a guide, considering controlled evaluation conditions specified in standards like EIA-809 or EIA/IS-535-BAAE, though some ambiguity may exist.
Factors Affecting Capacitor Ripple Current
Capacitance Value
Capacitance refers to a capacitor’s ability to store electrical charge. Higher capacitance capacitors have larger storage capacities and can therefore handle more ripple current. This is because they can absorb and release larger amounts of charge without significant voltage fluctuations.
Voltage Rating
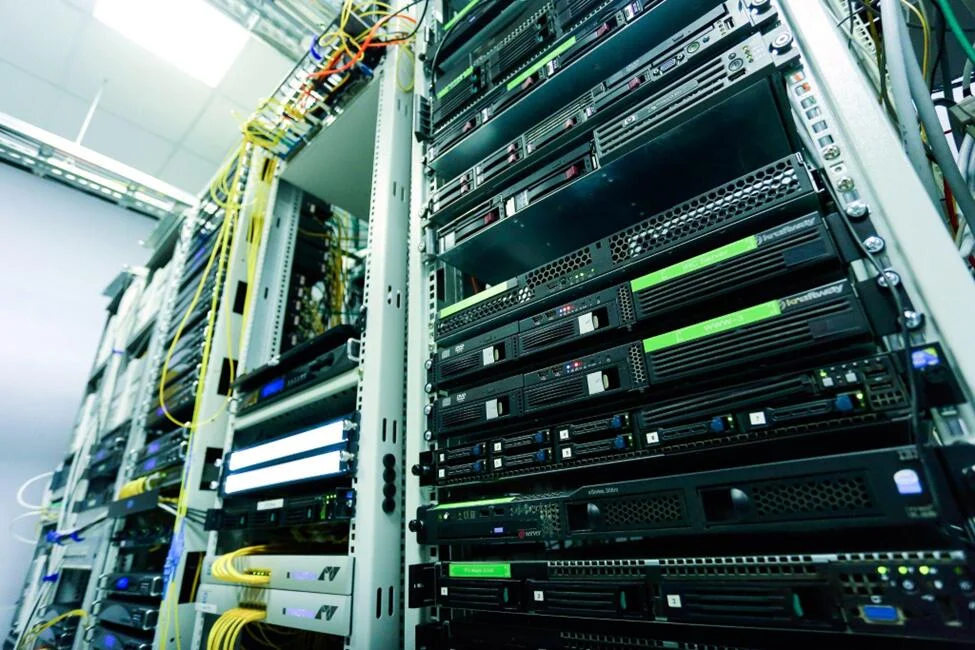
The voltage rating indicates the maximum voltage that a capacitor can safely withstand. Capacitors with higher voltage ratings can handle higher ripple currents without breakdown. Choosing a capacitor with an appropriate voltage rating ensures it can endure the voltage fluctuations present in the circuit without being damaged.
Frequency
Ripple current is directly proportional to the frequency of the alternating current or switching frequency in the circuit. Higher frequencies result in increased ripple currents. Capacitors must be selected to handle the expected frequency of the ripple current to prevent overheating and failure.
Temperature
Elevated temperatures decrease a capacitor’s ability to handle ripple current effectively. The heat generated by ripple currents can cause capacitors to degrade more quickly, leading to premature failure. Therefore, it’s crucial to consider the operating temperature range and ensure proper cooling mechanisms are in place to maintain capacitor reliability.
Factors 5 ESR (Equivalent Series Resistance)
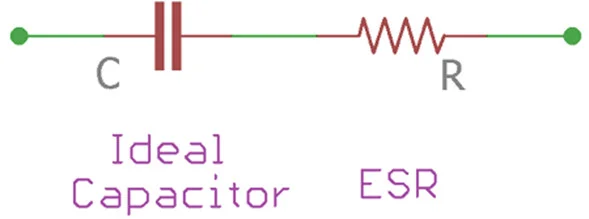
ESR is the internal resistance of a capacitor and represents the energy lost as heat when current flows through it. Lower ESR capacitors have more efficient heat dissipation capabilities, allowing them to handle higher ripple currents without overheating. Choosing capacitors with lower ESR values improves reliability and reduces the risk of failure.
Environmental Conditions
Environmental factors such as humidity, vibration, and altitude can impact a capacitor’s performance under ripple current conditions. Harsh environmental conditions can accelerate capacitor degradation and reduce their ability to handle ripple current effectively. Selecting capacitors rated for the specific environmental conditions of the application ensures reliable operation over time.
Component Aging
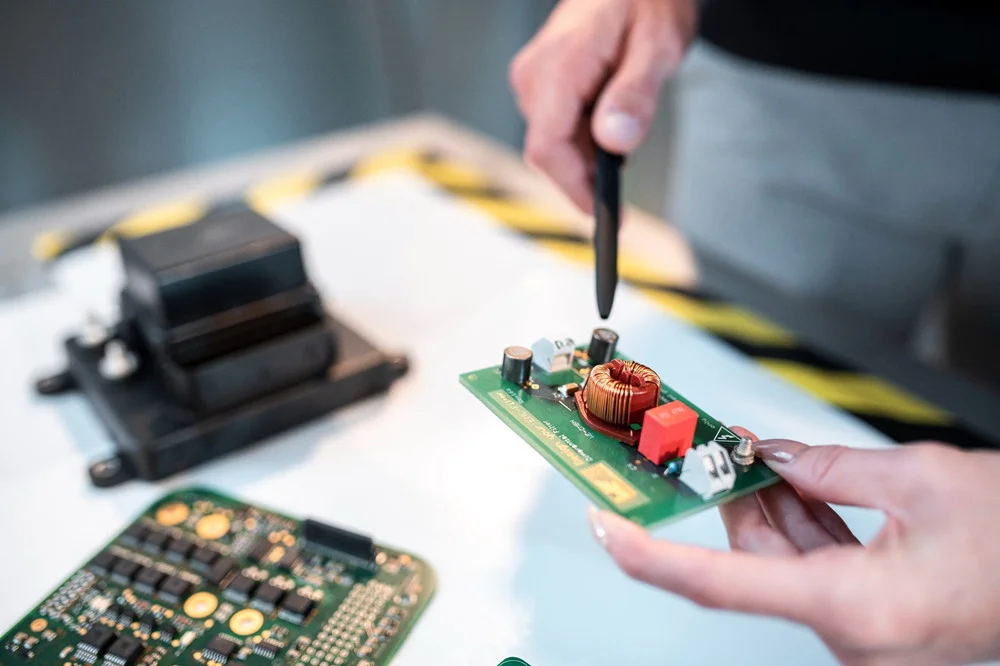
Over time, capacitors may degrade due to factors such as temperature, voltage stress, and environmental conditions. This degradation can reduce the capacitor’s ability to handle ripple current effectively, increasing the risk of failure. Monitoring capacitor aging and replacing aged components as needed is essential for maintaining circuit reliability.
Causes Of Capacitor Ripple Current
Voltage Fluctuations
Capacitor ripple current occurs when there are variations or fluctuations in the voltage levels across the capacitor. These fluctuations can be caused by changes in the input voltage, switching operations in the circuit, or other factors that affect the voltage waveform.
Frequency Of Operation
The frequency at which the circuit operates directly impacts the magnitude of the ripple current. Higher frequencies result in faster charging and discharging cycles of the capacitor, leading to increased ripple current. This is particularly significant in circuits with high-frequency switching operations, such as DC/DC converters and switching power supplies.
Capacitor Properties
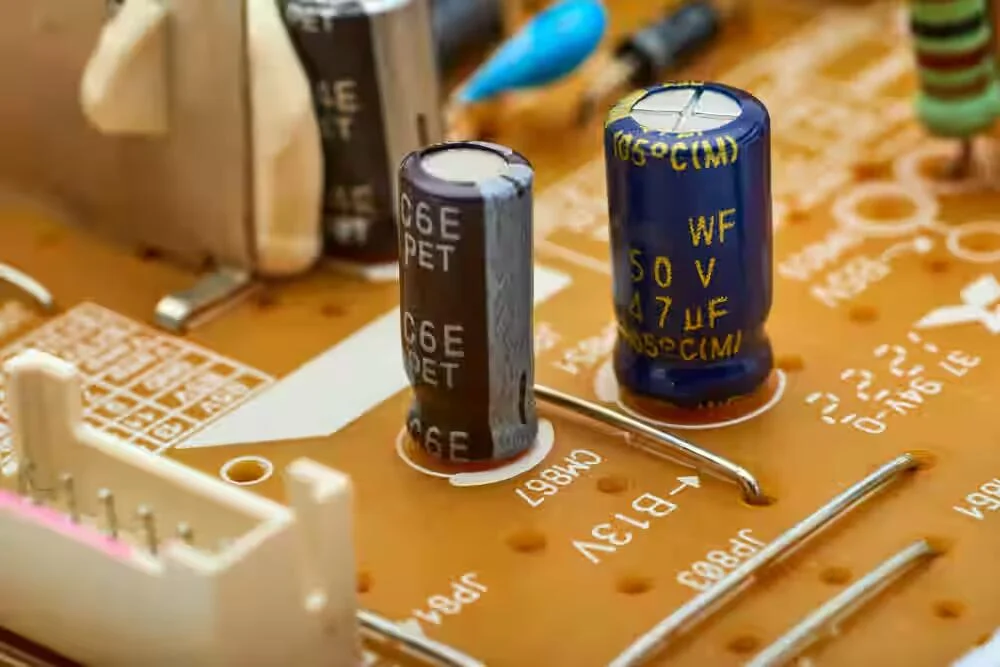
The properties of the capacitor itself play a crucial role in determining the magnitude of the ripple current. The capacitance value of the capacitor determines its ability to store and release charge, with higher capacitance capacitors being able to handle more ripple current.
Additionally, the Equivalent Series Resistance (ESR) of the capacitor affects its efficiency in dissipating heat generated by ripple current. Lower ESR capacitors can handle higher ripple currents more effectively.
Circuit Design
The design of the circuit, including the layout and configuration of components, can influence the flow of ripple current. Proper circuit design considerations, such as minimizing loop area and optimizing component placement, can help reduce the effects of ripple current on the overall system performance.
Environmental Conditions
Environmental factors such as temperature, humidity, and altitude can impact the performance of capacitors and contribute to the generation of ripple currents. High temperatures, for example, can increase the internal resistance of the capacitor and reduce its ability to handle ripple current effectively.
It’s important to consider the environmental conditions in which the circuit will operate and select capacitors accordingly to ensure reliable performance.
Effects Of Capacitor Ripple Current
Voltage Ripple:
The presence of ripple current within a circuit can cause voltage fluctuations across the capacitor, thereby impacting the stability of the power supply output. Consequently, this voltage ripple may introduce unwanted noise into delicate electronic systems. Additionally, it can potentially trigger malfunctions or errors during circuit operation.
Heating:
As ripple current flows through the capacitor, resistive heating occurs due to its internal resistance. This heating elevates the capacitor’s temperature, potentially surpassing its rated limits and shortening its lifespan. Moreover, excessive heating may trigger thermal runaway, resulting in the capacitor’s catastrophic failure.
Component Stress:
Elevated ripple currents exert pressure on the internal components of the capacitor, encompassing the dielectric material and electrodes. As a result, extended exposure to ripple current gradually deteriorates the capacitor’s performance. This degradation manifests as heightened Equivalent Series Resistance (ESR) and diminished capacitance.
Reduced Efficiency:
The presence of ripple currents within power supply circuits can diminish their efficiency by amplifying power losses within the capacitor. Consequently, this situation leads to energy wastage and a decline in the overall efficiency of the system. This effect is particularly pronounced in high-power applications where minimizing losses is of paramount importance.
Electromagnetic Interference (EMI):
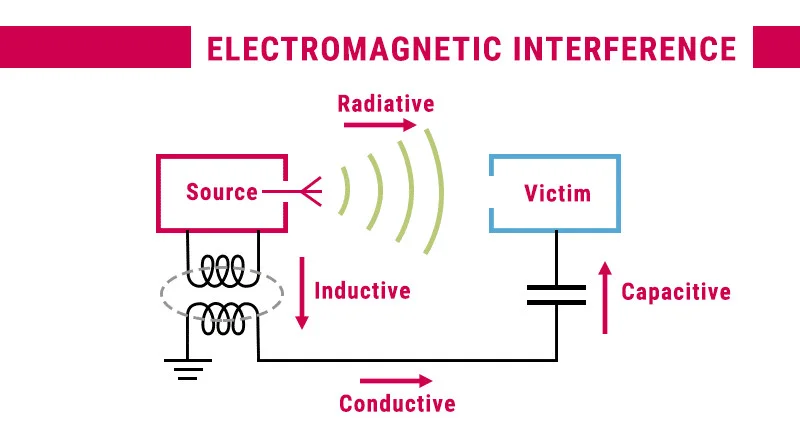
The occurrence of ripple current has the potential to generate electromagnetic interference (EMI) in adjacent components and circuits. As a result, this interference can cause signal distortion or disruption. Such occurrences have adverse effects on the performance of delicate electronic devices and communication systems, ultimately compromising their reliability and functionality.
Voltage Stress:
Elevated ripple currents pose a significant risk to the capacitor, particularly in scenarios characterized by rapid voltage transitions or high-frequency switching. In such instances, the capacitor may experience excessive voltage stress, surpassing its voltage rating and potentially resulting in breakdown or insulation failure.
Solutions To Mitigate Capacitor Ripple Current
Solution 1 Increase Capacitance
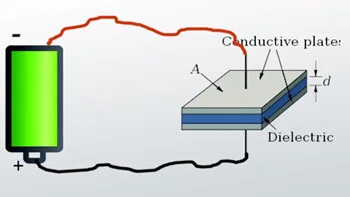
Capacitors with higher capacitance play a vital role in stabilizing voltage within circuits, particularly evident in AC to DC power supplies where ripple currents occur due to charging and discharging. Increased capacitance enables capacitors to store more charge, thereby absorbing surplus current during charging phases and releasing stored energy to counteract voltage drops.
This capability effectively reduces current fluctuations, resulting in a more consistent output voltage essential for maintaining the optimal performance of sensitive electronic devices.
Solution 2 Low ESR Capacitors
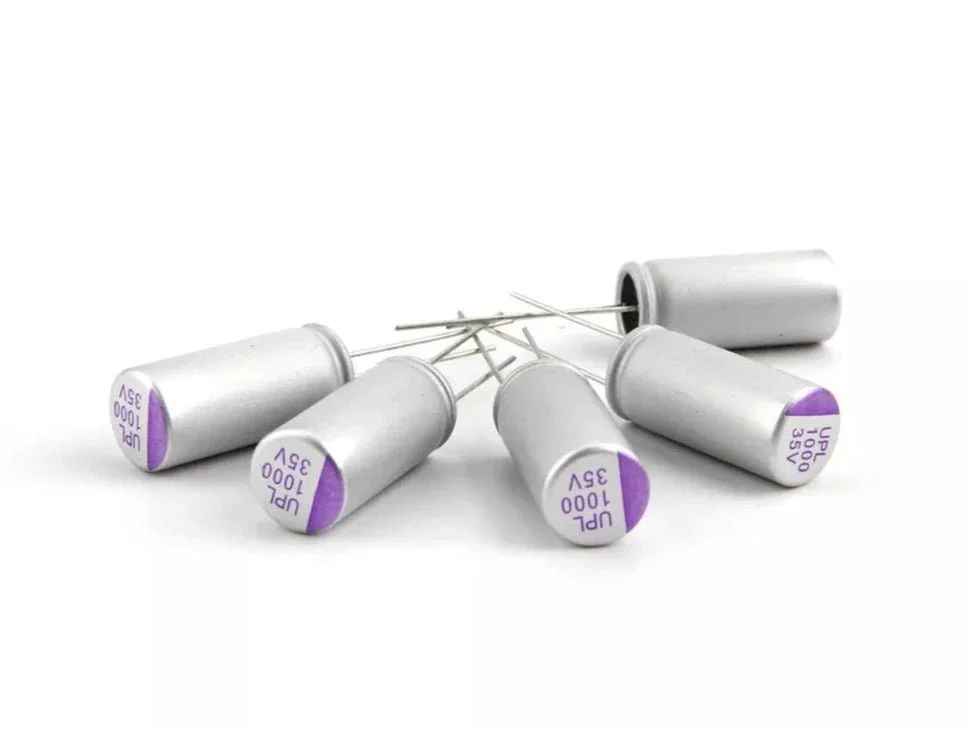
Capacitors’ electrostatic resistance (ESR) causes energy loss and heating, contributing to ripple currents in circuits. Choosing capacitors with low ESR reduces energy loss and heating, minimizing ripple currents, stabilizing voltage output, and enhancing circuit efficiency, crucial for reliable electronic systems.
Solution 3 Parallel Capacitors
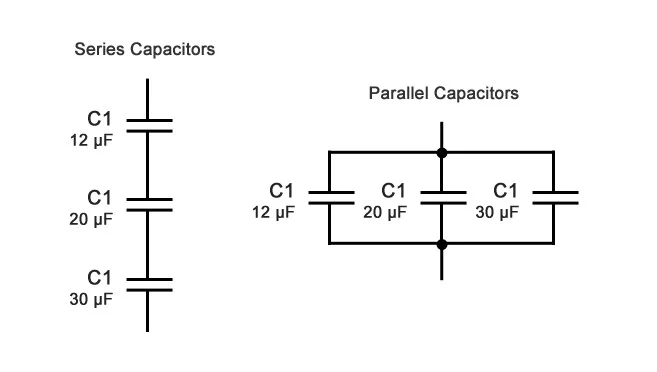
When capacitors are connected in parallel, their individual capacitances add up to create a larger total capacitance. This means that the combined capacitance of the parallel capacitors is greater than the capacitance of any single capacitor in the arrangement.
By increasing the total capacitance in the circuit, connecting capacitors in parallel helps to reduce the overall ripple current. With more capacitance available to store and smooth out charge variations, the effect of ripple currents on the circuit’s voltage output is diminished.
Distributing the load across multiple capacitors in parallel enables them to share the current more evenly. As a result, each capacitor experiences less stress and operates more efficiently, contributing to a reduction in ripple voltage across the load.
The parallel arrangement of capacitors provides redundancy and improves the stability of the circuit. In the event that one capacitor fails or becomes compromised, the others can continue to function, ensuring uninterrupted operation and minimizing the risk of voltage fluctuations.
Solution 4 Active Ripple Current Control
Integrating active circuits or employing control methods to manage ripple current is an effective strategy for mitigating its impacts. This approach may entail the utilization of feedback loops or control algorithms, which dynamically adjust the charging and discharging rates of capacitors.
Solution 5 Temperature Control
Ripple current increases with temperature due to increased ESR and decreased capacitance. Proper thermal management techniques, such as heat sinking or airflow, can help maintain stable capacitor performance and reduce ripple currents.
Solution 6 Voltage Regulation
Utilizing voltage regulation circuits or voltage regulators downstream of the capacitor can help compensate for variations in input voltage and reduce the impact of ripple current on the output voltage.
Solution 7 Layout And Routing
Proper circuit layout and routing techniques can minimize parasitic resistance and inductance, which contribute to ripple current. Short, direct traces and low-resistance connections help improve capacitor performance.
Solution 8 Filtering Techniques
Incorporating additional passive filtering components, such as inductors and resistors, in conjunction with capacitors can further reduce ripple current by creating low-pass filter networks that attenuate high-frequency components.
Conclusion
Comprehending and effectively addressing capacitor ripple current is paramount in electrical engineering to uphold peak system performance and reliability. The emergence of ripple currents, stemming from the charging and discharging cycles of capacitors, poses a risk of voltage fluctuations, which can disrupt circuits, particularly in mission-critical scenarios.
This article delves into the nuances of capacitor ripple current, uncovering its origins, its effects on circuits, and tactics for mitigation. By unraveling this complexity, readers can glean valuable insights to optimize electronic system performance and durability across diverse applications.