Ever wondered how a simple device can store electrical energy?
The heart of a capacitor lies in its dielectric material. This often-overlooked component plays a crucial role in determining a capacitor’s performance and efficiency.
In this blog post, we’ll delve into the world of dielectric materials, exploring what they are, how they work, and why they’re essential to the functioning of capacitors.
So, let’s unravel the mystery of the capacitor dielectric and discover the secrets behind its energy-storing capabilities.
What Is Capacitor Dielectric
A capacitor dielectric is an insulating material placed between the two conductive plates of a capacitor. It plays a crucial role in determining the capacitor’s capacitance, voltage rating, and overall performance.
Capacitor Dielectric Material
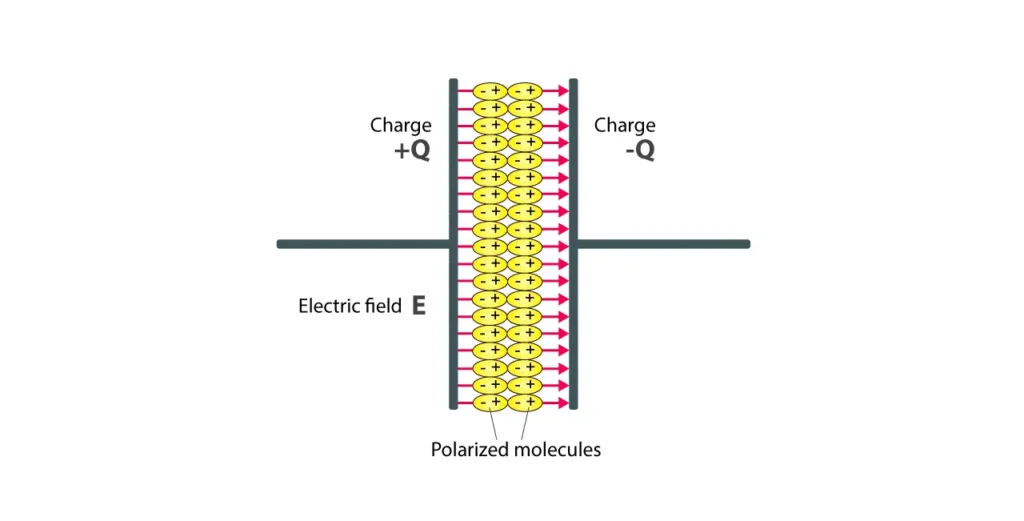
A dielectric material is an insulating substance placed between the two conductive plates of a capacitor. It plays a crucial role in determining the capacitor’s capacitance, voltage rating, and overall performance.
Common types of dielectric materials:
Ceramic:
- Types: C0G (NP0), X7R, Y5V, Z5U3
- Characteristics: High stability, high capacitance, high temperature stability (C0G), lower temperature stability but higher capacitance (X7R, Y5V, Z5U)
- Applications: Filters, oscillators, timing circuits, decoupling capacitors4
Film:
- Types: Polypropylene, Polyester (Mylar), Polystyrene5
- Characteristics: High stability, low loss, high voltage rating6
- Applications: Timing circuits, filters, coupling capacitors
Electrolytic:
- Types: Aluminum Electrolytic, Tantalum Electrolytic7
- Characteristics: High capacitance in a small package, polarized8
- Applications: Power supply filtering, energy storage9
Air:
- Characteristics: Low capacitance, high voltage rating, low loss
- Applications: Variable capacitors, high-frequency circuits10
Vacuum:
- Characteristics: Extremely high voltage rating, low loss, high stability
- Applications: High-voltage applications, such as power transmission and particle accelerators
The choice of dielectric material depends on the specific requirements of the application, such as capacitance, voltage rating, temperature stability, frequency response, and cost.
Key properties of a dielectric material:
- Permittivity: A measure of the dielectric’s ability to store electrical energy. A higher permittivity leads to a higher capacitance.11
- Dielectric Strength: The maximum electric field that the dielectric can withstand before breaking down and conducting electricity.12
- Temperature Stability: The dielectric’s ability to maintain its properties over a wide range of temperatures.13
- Frequency Response: The dielectric’s performance at different frequencies, especially important for high-frequency applications.14
By understanding the role of the dielectric, you can select the right capacitor for your specific application.
Capacitor Dielectric Types
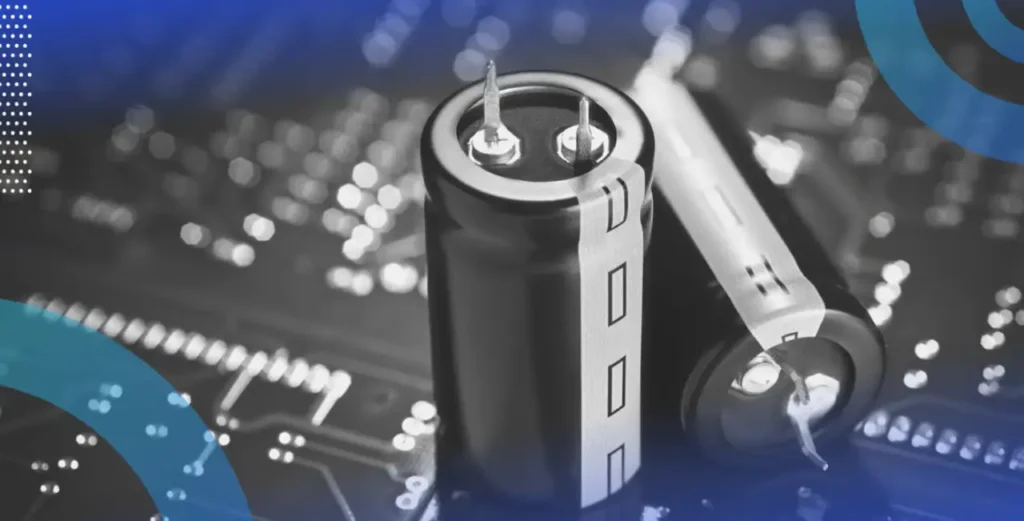
Here are some common types of capacitor dielectrics:
1. Ceramic Dielectric:
- Types: C0G (NP0), X7R, Y5V, Z5U
- Characteristics: High stability, high capacitance, high temperature stability (C0G), lower temperature stability but higher capacitance (X7R, Y5V, Z5U)
- Applications: Filters, oscillators, timing circuits, decoupling capacitors
2. Film Dielectric:
- Types: Polypropylene, Polyester (Mylar), Polystyrene
- Characteristics: High stability, low loss, high voltage rating
- Applications: Timing circuits, filters, coupling capacitors
3. Electrolytic Dielectric:
- Types: Aluminum Electrolytic, Tantalum Electrolytic
- Characteristics: High capacitance in a small package, polarized
- Applications: Power supply filtering, energy storage
4. Air Dielectric:
- Characteristics: Low capacitance, high voltage rating, low loss
- Applications: Variable capacitors, high-frequency circuits
5. Vacuum Dielectric:
- Characteristics: Extremely high voltage rating, low loss, high stability
- Applications: High-voltage applications, such as power transmission and particle accelerators
The choice of dielectric material depends on the specific requirements of the application, such as capacitance, voltage rating, temperature stability, frequency response, and cost.
Capacitor With Multiple Dielectrics
A capacitor with multiple dielectrics is a variation of the standard parallel-plate capacitor where the space between the plates is filled with two or more different dielectric materials. This configuration can offer unique properties and applications.
Series Configuration
When two dielectrics are placed in series between the plates, the equivalent capacitance can be calculated using the formula for capacitors in series:
1/C_eq = 1/C_1 + 1/C_2
Where:
C_eq
is the equivalent capacitanceC_1
andC_2
are the capacitances of the two capacitors with individual dielectrics
Parallel Configuration
When two dielectrics are placed in parallel, the equivalent capacitance can be calculated using the formula for capacitors in parallel:
C_eq = C_1 + C_2
Mixed Configurations
More complex configurations involving multiple dielectrics in series and parallel combinations can be analyzed using the equivalent capacitance formulas for these configurations.
Key Considerations for Capacitors with Multiple Dielectrics:
- Dielectric Strength: The overall voltage rating of the capacitor is limited by the dielectric with the lowest breakdown voltage.
- Capacitance: The effective capacitance depends on the dielectric constants and thicknesses of the individual dielectrics.
- Temperature Stability: The temperature stability of the capacitor is influenced by the temperature coefficients of the different dielectrics.
- Frequency Response: The frequency response of the capacitor can be affected by the dielectric properties, particularly at higher frequencies.
Applications:
Capacitors with multiple dielectrics can be used in various applications, including:
- High-voltage capacitors: By using multiple layers of dielectric material, it is possible to achieve higher voltage ratings.
- Temperature-stable capacitors: By combining dielectrics with different temperature coefficients, it is possible to create capacitors with improved temperature stability.
- High-frequency capacitors: By using low-loss dielectric materials, it is possible to create capacitors that can operate at high frequencies with minimal energy dissipation.
By carefully selecting the types and thicknesses of the dielectric materials, engineers can design capacitors with specific electrical properties to meet the requirements of various electronic circuits.
Spherical Capacitor With Dielectric
A spherical capacitor consists of two concentric spherical conductors separated by a dielectric material. The dielectric material, with its high permittivity, significantly enhances the capacitance of the capacitor compared to a similar configuration without a dielectric.
Capacitance Formula
The capacitance of a spherical capacitor with a dielectric material filling the space between the conductors is given by:
C = 4πε₀εᵣ * (ab) / (b-a)
Where:
- C is the capacitance in Farads (F)
- ε₀ is the permittivity of free space (approximately 8.85 × 10⁻¹² F/m)
- εᵣ is the relative permittivity (dielectric constant) of the material
- a is the radius of the inner sphere
- b is the radius of the outer sphere
Key Points:
- Dielectric Effect: The dielectric material increases the capacitance by reducing the electric field between the conductors.
- Geometric Factors: The capacitance is influenced by the radii of the inner and outer spheres.
- Dielectric Constant: A higher dielectric constant leads to a higher capacitance.
Applications of Spherical Capacitors
While less common than parallel-plate capacitors, spherical capacitors have specific applications:
- High-voltage applications: Their geometry allows for higher voltage ratings.
- Calibration standards: They can be used as precision standards for capacitance measurements.
- Specialized electronic circuits: In certain niche applications where their unique geometry offers advantages.
By understanding the principles of spherical capacitors and the role of dielectric materials, engineers can design and utilize these components effectively in various electronic systems.
How Does Capacitor Dielectric Work
A capacitor dielectric works by increasing the capacitance of a capacitor while reducing the electric field strength between the plates.
Here’s a breakdown of the process:
- Polarization: When a voltage is applied across the capacitor’s plates, an electric field is created. This electric field causes the molecules within the dielectric material to polarize.
- Induced Charges: The polarization of the dielectric material induces charges on its surface. These induced charges are opposite in sign to the charges on the capacitor plates.
- Reduced Electric Field: The induced charges on the dielectric’s surface partially counteract the electric field between the plates, resulting in a weaker net electric field.
- Increased Capacitance: A weaker electric field means that a higher amount of charge can be stored on the plates for a given voltage. This effectively increases the capacitance of the capacitor.
Electric Field in a Capacitor With Dielectric
The introduction of a dielectric material between the plates of a capacitor reduces the electric field strength.
How it works:
- Polarization: When a voltage is applied across the capacitor plates, an electric field is created. This electric field polarizes the dielectric material, causing its molecules to align with the field.
- Induced Charges: The polarization of the dielectric induces charges on its surface, opposite in sign to the charges on the capacitor plates.
- Reduced Electric Field: These induced charges partially counteract the electric field between the plates, resulting in a weaker net electric field.
Mathematical Representation:
The electric field strength (E) in a parallel-plate capacitor without a dielectric is given by:
E = σ / ε₀
where:
E
is the electric field strengthσ
is the surface charge densityε₀
is the permittivity of free space
When a dielectric with a relative permittivity (dielectric constant) of εᵣ is inserted between the plates, the electric field strength becomes:
E = σ / (ε₀ * εᵣ)
As you can see, the electric field strength is reduced by a factor of εᵣ.
Key points to remember:
- Reduced Electric Field: The dielectric reduces the electric field strength between the plates.
- Increased Capacitance: The reduced electric field allows for a higher amount of charge to be stored on the plates for a given voltage, increasing the capacitance.
- Dielectric Constant: The dielectric constant (εᵣ) of the material determines the extent to which the electric field is reduced and the capacitance is increased.
By understanding the effect of a dielectric on the electric field, we can optimize the performance of capacitors for various applications.
Capacitor With Dielectric Formula
The formula for the capacitance of a parallel-plate capacitor with a dielectric material between the plates is:
C = ε₀ * εᵣ * A / d
Where:
- C is the capacitance1 in Farads (F)
- ε₀ is the permittivity of free space (approximately 8.85 × 10⁻¹² F/m)2
- εᵣ is the relative permittivity (dielectric constant) of the material3
- A is the area of overlap of the plates in square meters (m²)
- d is the distance between the plates in meters (m)
Key points to remember:
- Dielectric Constant (εᵣ): This dimensionless quantity represents the factor by which the capacitance is increased compared to a capacitor with a vacuum as the dielectric.4 Different dielectric materials have different dielectric constants.5
- Increased Capacitance: The introduction of a dielectric material between the plates increases the capacitance of the capacitor.6
- Reduced Electric Field: The dielectric material reduces the electric field between the plates for a given voltage, allowing for higher voltage ratings.
By understanding this formula and the properties of dielectric materials, you can select the appropriate capacitor for various electronic applications.
Capacitance of Capacitor With Dielectric
Here is the capacitance of parallel plate capacitor with dielectric as follows:
The capacitance of a parallel-plate capacitor with a dielectric material between the plates is given by the formula:
C = ε₀ * εᵣ * A / d
Where:
- C is the capacitance in Farads (F)
- ε₀ is the permittivity of free space (approximately 8.85 × 10⁻¹² F/m)
- εᵣ is the relative permittivity (dielectric constant) of the material
- A is the area of overlap of the plates in square meters (m²)
- d is the distance between the plates in meters (m)
The dielectric constant, εᵣ, is a dimensionless quantity that represents the factor by which the capacitance is increased compared to a capacitor with a vacuum as the dielectric. Different dielectric materials have different dielectric constants.
By introducing a dielectric material between the plates, the capacitance of the capacitor increases. This is because the dielectric material reduces the electric field between the plates for a given voltage, allowing for a higher amount of charge to be stored on the plates.
Capacitance of Cylindrical Capacitor With Dielectric
For a cylindrical capacitor with a dielectric material between the conductors, the capacitance per unit length is given by:
C/L = (2πε₀εᵣ) / ln(b/a)
Where:
- C/L is the capacitance per unit length in Farads per meter (F/m)
- ε₀ is the permittivity of free space (approximately 8.85 × 10⁻¹² F/m)
- εᵣ is the relative permittivity (dielectric constant) of the material
- b is the radius of the outer conductor
- a is the radius of the inner conductor
This formula shows that the capacitance of a cylindrical capacitor increases with the dielectric constant of the material, the length of the capacitor, and the ratio of the outer and inner radii.
It’s important to note that this formula assumes a uniform dielectric material filling the space between the conductors. If the dielectric is non-uniform or has variations in its properties, the calculation becomes more complex.
What Does a Dielectric Do to a Capacitor
A dielectric material, when inserted between the plates of a capacitor, significantly increases its capacitance.
Here’s how it works:
- Polarization: When a voltage is applied across the capacitor plates, an electric field is created. This electric field polarizes the dielectric material, causing its molecules to align with the field.
- Induced Charges: The polarization of the dielectric induces charges on its surface, opposite in sign to the charges on the capacitor plates.
- Reduced Electric Field: These induced charges partially counteract the electric field between the plates, resulting in a weaker net electric field.
- Increased Capacitance: A weaker electric field means that a higher amount of charge can be stored on the plates for a given voltage. This effectively increases the capacitance of the capacitor.
Key benefits of using a dielectric:
- Increased Capacitance: A dielectric allows for a higher capacitance in a smaller physical size.
- Higher Voltage Rating: The dielectric can withstand higher voltages before breaking down.
- Improved Performance: Different dielectric materials offer various properties, such as temperature stability, frequency response, and energy density.
By understanding the role of the dielectric, we can select the appropriate capacitor for specific applications, optimizing performance and efficiency in electronic circuits.
Capacitor Dielectric Absorption
Dielectric absorption is a phenomenon in capacitors where, after being discharged, they gradually regain some of their original voltage. This is due to the slow release of stored energy from the dielectric material.
How it happens:
- Polarization: When a capacitor is charged, the electric field polarizes the dielectric material, aligning its molecules.
- Charge Trapping: Some of these polarized molecules become trapped, unable to immediately realign when the capacitor is discharged.
- Delayed Release: Over time, these trapped charges gradually release their energy, causing the capacitor’s voltage to rise again.
Impact on Capacitor Performance:
- Voltage Spikes: In circuits with high-speed switching, dielectric absorption can cause voltage spikes, potentially damaging sensitive components.
- Timing Errors: In timing circuits, dielectric absorption can introduce inaccuracies.
- Signal Integrity: In high-speed digital circuits, dielectric absorption can degrade signal integrity.
Mitigation Techniques:
- Low Dielectric Absorption Materials: Using materials with low dielectric absorption, such as polypropylene or polystyrene, can minimize the effect.
- Short Discharge Time: Discharging the capacitor quickly can reduce the amount of charge trapped in the dielectric.
- Multiple Discharge Cycles: Repeatedly discharging and charging the capacitor can help release trapped charges.
While dielectric absorption is a common characteristic of capacitors, its impact can be mitigated through careful component selection and circuit design. Understanding this phenomenon is essential for designing reliable and accurate electronic circuits.
How Do Dielectrics Affect Capacitors
Dielectrics are insulating materials that significantly impact the performance of capacitors. When inserted between the plates of a capacitor, a dielectric material:
Increases Capacitance:
- Polarization: The dielectric material polarizes when an electric field is applied across the capacitor plates. This polarization induces opposite charges on the dielectric’s surface, facing the capacitor plates.
- Reduced Electric Field: These induced charges partially counteract the electric field between the plates, resulting in a weaker net electric field.
- Increased Charge Storage: With a weaker electric field, the capacitor can store more charge on its plates for a given voltage, leading to an increase in capacitance.
Increases Voltage Rating:
- Dielectric Strength: Dielectric materials have a specific dielectric strength, which is the maximum electric field they can withstand before breaking down and conducting electricity.
- Higher Voltage Tolerance: By using dielectrics with higher dielectric strengths, capacitors can handle higher voltages without breaking down.
Improves Performance:
- Reduced Leakage Current: Dielectric materials can reduce leakage current, improving the capacitor’s efficiency and lifespan.
- Enhanced Temperature Stability: Some dielectric materials exhibit excellent temperature stability, ensuring consistent performance across a wide range of temperatures.
- Improved Frequency Response: Certain dielectrics are optimized for high-frequency applications, minimizing energy loss.
In summary, dielectrics play a crucial role in enhancing the performance of capacitors by:
- Increasing capacitance
- Improving voltage rating
- Reducing leakage current
- Enhancing temperature stability
- Optimizing frequency response
By understanding the impact of dielectrics, engineers can select the appropriate capacitor for specific applications, optimizing performance and efficiency in electronic circuits.
Conclusion
Capacitors, with their ability to store electrical energy, are fundamental components in countless electronic devices. The dielectric material, sandwiched between the capacitor’s conductive plates, plays a crucial role in determining its capacitance, voltage rating, and overall performance.
Key takeaways:
- Dielectric Material: The type of dielectric material used significantly impacts a capacitor’s properties.
- Capacitance: The capacitance of a capacitor is directly influenced by the dielectric’s permittivity.
- Voltage Rating: The dielectric strength determines the maximum voltage a capacitor can withstand.
- Temperature Stability: The dielectric material’s stability over temperature variations is crucial for reliable performance.
By understanding the role of the dielectric, you can make informed choices when selecting capacitors for your electronic projects.
Need high-quality capacitors?
Consider Weishi Electronics, a trusted manufacturer offering a wide range of capacitors to meet your diverse needs. From ceramic capacitors to electrolytic capacitors, Weishi provides reliable and efficient solutions for your electronic projects.